El Niño events are one of the “most spectacular instances of interannual variability in the ocean” with “profound consequences for climate and the ocean ecosystem” (Cane 1986). Perturbations in the atmosphere directly influence the ocean with long-term effects on environmental variability in the tropical Pacific Ocean as the El Niño-Southern Oscillation (ENSO) shifts between El Niño, neutral, and La Niña states on a timescale of two to seven years. On longer timescales, teleconnections from the tropics to extratropical regions drive Pacific decadal variability, and these can be both oceanic and atmospheric in nature. Mid-latitude variability of the Pacific Decadal Oscillation (PDO) has been associated with ENSO (e.g., Newman et al. 2003) and is distinguished from ENSO in part by its multidecadal timescale (20-30 years; 50 years). The PDO is dependent upon ENSO as a response to the combined effects of atmospheric noise (Newman et al. 2003), as well as the asymmetry of the ENSO cycle (Rodgers et al. 2004). Therefore, when discussing decadal variability of the northeast Pacific, we are referring to the delayed impacts of ENSO.
Ecosystem impacts of northeast Pacific variability
Given the complex influence of tropical climate on northeast Pacific ecosystems, there is significant overlap between ENSO signals and higher frequency modes of the PDO Index. It is widely recognized that interactions between these two climate modes drive substantial ecosystem variability on a range of time and space scales. Large regime shifts in the North Pacific that have reverberated throughout the ecosystem, from physics to fish, are recurring patterns now associated with low-frequency changes in sea surface temperature (SST) that characterize the PDO (e.g., Mantua et al. 1997). Some of the higher-frequency fluctuations in ecosystem variables of the northeast Pacific that have not been successfully attributed to PDO or ENSO are now thought to be driven by an intermediate mode of variability called the North Pacific Gyre Oscillation (NPGO). While the PDO is characterized as the first empirical orthogonal function (EOF) of SST, NPGO is defined as the first EOF of both SST and sea surface height (SSH) anomalies (Di Lorenzo et al. 2008). Compared to PDO and ENSO, NPGO is more closely tied to variations in salinity, nutrient upwelling, and chlorophyll a (chl-a) in the long-running California Cooperative Oceanic Fisheries Investigations (CalCOFI) time-series. DiLorenzo et al. (2008) suggest that major ecosystem regime shifts require a simultaneous and opposite sign reversal of the NPGO and PDO, as was seen shortly after the massive ENSO event of 1997/98, and all three indices relate back to dynamics in the tropical Pacific. The struggle is to understand how these low- to high(er)-frequency modes of variability in the climate and physics drive fluctuations in biogeochemistry and coastal ecology.
As discussed by Jacox et al. (this post), there is an expected or canonical set of physical conditions associated with ENSO in the California Current System (CCS). This physical response to ENSO generally includes: 1) changes in surface wind stress that alter the strength of coastal upwelling and downwelling; 2) remote oceanic forcing by coastally trapped waves that propagate poleward along the US West Coast and modify thermocline depth and coastal stratification; and 3) changes to alongshore advection (Jacox et al., this post). The ecological response of the coastal marine environment includes changes in primary production and the community composition of plankton and higher trophic levels that can be directly or more subtly related to these physical factors. Primary production is driven by vertical nutrient flux to well-lit surface waters; nutrient supply is related to upwelling magnitude, upwelling source depth, and nutrient concentrations at the source depth. ENSO-related processes are also important for interannual and seasonal variability of oxygen concentrations and carbonate biogeochemistry on the Washington and Oregon Shelves (Siedlecki et al. 2015). In this article, we highlight some of the modeling and observational studies that have successfully attributed ENSO-like variability to specific impacts on the biogeochemistry and lower-trophic level organisms of the northeast Pacific and CCS.
Carbon dioxide
Numerical models are widely employed to diagnose climatic forcing of the physical and biogeochemical conditions of the northeast Pacific. For instance, using a fully coupled ocean and biogeochemical model, Xiu and Chai (2014) found that after accounting for atmospheric effects, the air-sea flux and resulting pCO2 of sea water in the Pacific was significantly correlated (0.6) to the Multivariate ENSO Index (MEI) with a lag of ten months. Similarly, Wong et al. (2010) found that sea surface pCO2 was significantly correlated with the MEI in the northeast Pacific. Biogeochemical models of different complexity have also highlighted the connection between PDO and the interannual variability of air-sea CO2 fluxes (e.g., McKinley et al. 2006). These studies also demonstrate that the individual components controlling surface ocean pCO2 in the northeast Pacific respond to PDO with significant amplitudes, but that their combined influence has a relatively small effect on the CO2 fluxes in this region. Xiu and Chai (2014) showed that the dominant driver of North Pacific pCO2 variability is anthropogenic CO2, whereas air-sea CO2 flux is more closely correlated with the PDO and the NPGO.
Nutrients and chlorophyll
In the coastal regions of the northeast Pacific, such as the CCS, ENSO significantly impacts the nutrient supply due to modifications of upwelling and source waters mentioned above (Jacox et al., this post). At the peak of the El Niño season in December-January, Frischknecht et al. (2016) found a pattern in the development of chlorophyll events through a modeling study focused on the CCS. Around the onset of the El Niño year, chlorophyll anomalies were consistently low. This pattern was even more pronounced during the spring of the following year. In spring of the second year (i.e. with the onset of the upwelling season), all events shared the development of a strong negative chlorophyll anomaly. Frischknecht et al. (2016) attributed this phenomenon to a persistent lack of nutrients to support production driven by a combination of physical mechanisms impacting the thermocline (Jacox et al. 2015; 2016; this post) and light limitation at the onset of the upwelling season. Consequently, El Niño events disrupt the biogeochemical cycling in these systems for months, even years, after the event is over. The observations in Oregon, from the Newport line in Fisher et al. (2015), detail the nitrate anomalies from 1995 to 2015, and the nitrate anomalies remain negative long after the Niño-3.4 SST anomaly suggested that the event was over. This may contribute to the success surrounding seasonal forecast systems like J-SCOPE, in which forecasts of biogeochemical parameters (e.g., bottom oxygen) outperformed those of physical variables (e.g., SST) in terms of predictive skill (Siedlecki et al. 2016).
Oxygen and carbon
The relationship between ENSO and nutrient availability from source waters can serve as an analog for oxygen and carbon content. We would expect from observed stoichiometry that when nutrients are low, oxygen is relatively high and carbon is low. In California, this has been documented: El Niño events correlate to higher oxygen and pH, while La Niña events are correlated with lower oxygen and pH (e.g., Nam et al. 2011). In the northern CCS along the Washington and Oregon coasts, the interannual variability in oxygen content of source waters has been correlated to NPGO more than ENSO (Peterson et al. 2013). Consistent with these findings, oxygen has been increasing since 2010 and aragonite saturation state (a measure of the availability of carbonate ion to calcifying organisms) has been elevated in 2015-2016 relative to the year prior in both Oregon and California (McClatchie et al. 2016).
Primary production & particle export
As an eastern boundary upwelling region, the CCS is among the most productive in the world in terms of primary production and fisheries. The suppression of nutrient availability described above can be thought of as reduced “upwelling efficacy” that leads to reduced primary production in the CCS, while La Niña often has the opposite effect due to associated increases in the upwelling efficacy (Jacox et al. 2015). In the southern CCS, the 1997/1998 El Niño led to a significant deepening of the nutricline, with the strongest effects along CalCOFI Line 80, and a pronounced regional reduction of primary production (Bograd and Lynn 2001). The uptake of silicon increased in central California (Santa Barbara Channel) during the onset of the 1997 event, suggesting that diatoms were major drivers of the primary productivity prior to the 1998 spring season when overall productivity was reduced in response to density surface adjustments (Shipe and Brzezinski 2003). Despite reductions in surface layer primary productivity in response to the El Niño, export ratios of particulate organic carbon and particulate organic nitrogen increased during the spring of 1998 relative to the 1994-1997 period, while biogenic Si flux decreased in response to the El Niño (Shipe et al. 2002). This counterintuitive result appears to be due to an increase in particulate material exported to depth. By 1999, ratios of Si/N and Si/C had not recovered to pre-El Nino conditions.
Phytoplankton community composition
Warmer waters and changes in nutrient supply associated with ENSO can lead to phytoplankton community shifts such as an influx of coccolithophores or an increase in harmful algal blooms (HABs). The most common harmful algal bloom organism in the CCS is the diatom genus Pseudo-nitzschia. McCabe et al. (2016) recently observed a link between the Oceanic Niño Index (ONI), the Pseudo-nitzschia growth rate anomaly determined from temperature-growth relationships, and domoic acid levels in razor clams over a 16-year period (Figure 1), implicating El Niño-driven warming in the unprecedented 2015 HAB along the US West Coast. Similarly, McKibben et al. (2017) linked warm phases of the PDO and ONI to domoic acid in shellfish in the northern CCS. The toxic blooms off Newport in 2015 were the most prolonged (late-April through October 2015) and among the most toxic ever observed off Oregon (Du et al. 2015; McKibben et al. 2017). Conversely, Santa Barbara Basin sediment trap data showed no significant correlation between a 15-year record of domoic acid levels and PDO, NPGO, or ENSO indices; however, there was a strong change point in the frequency and toxicity of these blooms following the 1997/1998 ENSO (Sekula-Wood et al. 2011).
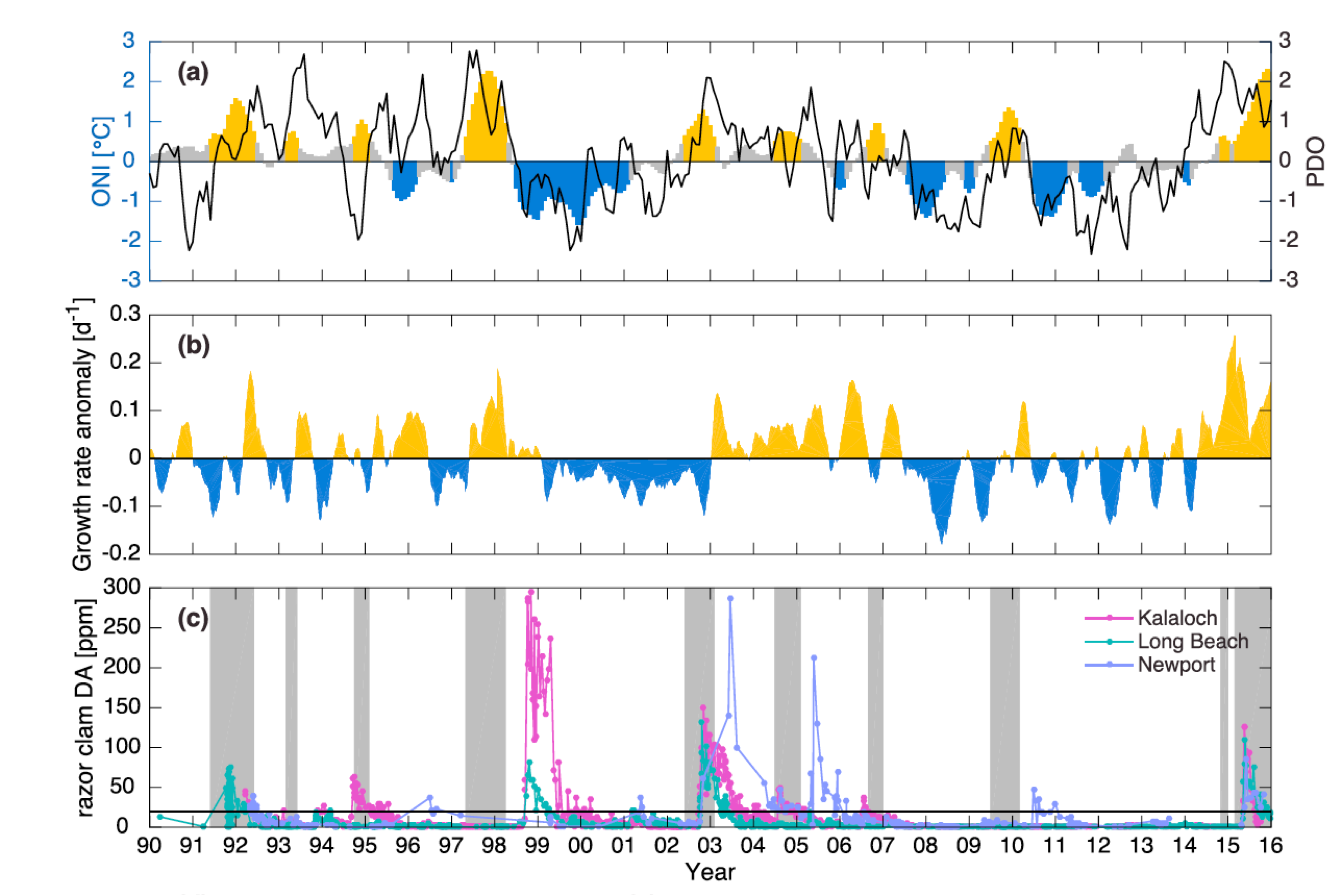
Figure 1. Records of razor clam toxicity and the potential growth rate anomaly for Pseudo-nitzschia spp. are plotted below the Oceanic Niño Index (gold = El Niño, blue = La Niña, gray = neutral) to illustrate the association between ENSO events and harmful algal blooms in the northern CCS. The potential for Pseudo–nitzschia growth does not always coincide with records of high domoic acid in shellfish, e.g., 1997 El Niño. Figure adapted from McCabe et al. (2016).
Zooplankton community composition
Off the Oregon coast, a 21-year time-series of fortnightly hydrography and plankton sampling of shelf and slope waters showed that the water masses (and thus the plankton) that dominate shelf and slope waters vary seasonally, interannually, and on decadal scales. Thus, it is a simple matter to track the timing of summer or winter arrival, ENSO events, and changes in sign of the PDO (Figure 2). During summer months, northerly winds drive surface waters offshore (Ekman transport), which are replaced by the upwelling of cold nutrient-rich waters that penetrate the continental shelf and fuel high primary production. Northerly winds also enhance the southward transport of water (and plankton) from the coastal Gulf of Alaska into the coastal northern CCS, and these species are referred to as ‘cold water’ or ‘northern species.’ During winter, the winds reverse and the poleward Davidson current transports warm coastal water from southern California to the northern CCS, bringing with it ‘southern species’ of plankton. On longer timescales (5-10 years), cold-water, northern copepods are largely replaced by warm-water, southern copepods during El Niño events (Fisher et al. 2015) and during the positive phase of the PDO (Keister et al. 2011). Incorporating the physiological response of these zooplankton groups into biogeochemical-ecosystem models (in addition to the effects of physical transport) will be essential for advancing our predictive capacity of plankton communities in the CCS.
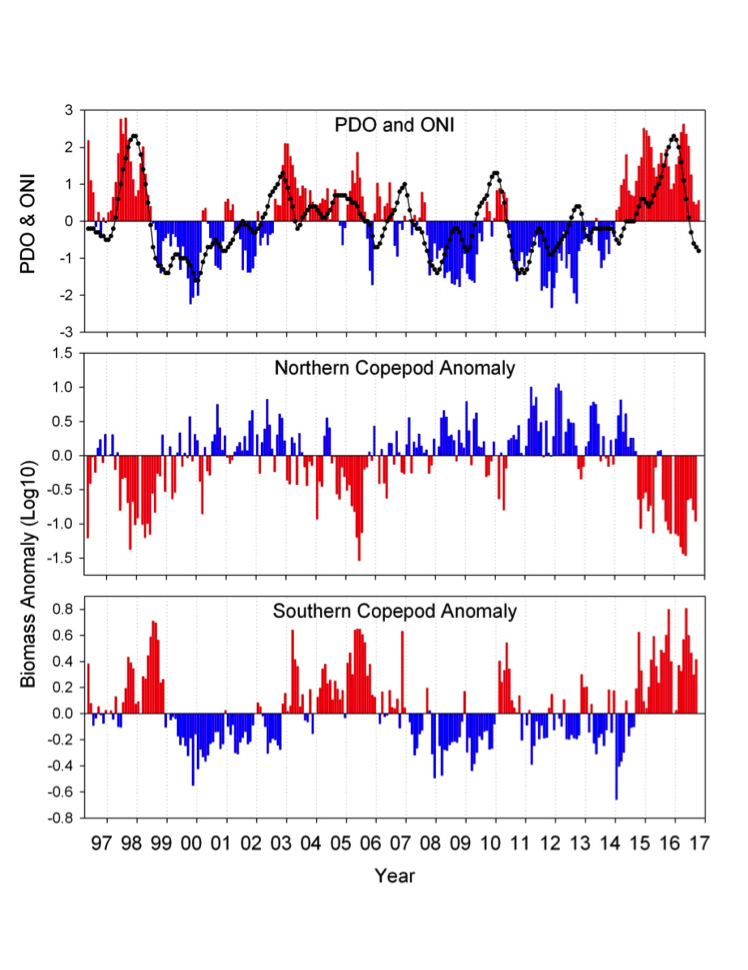
Figure 2. Monthly time series of the Pacific Decadal Oscillation and Oceanic Niño Index (upper) and monthly-averaged biomass anomalies of northern copepods (middle) and southern copepods (lower). Note the high coherence between the PDO and ONI with the copepod time series – positive anomalies of northern copepods are correlated with negative PDO and ONI; positive anomalies of southern copepods are correlated with positive PDO and ONI.
Predicting ecosystem response to ENSO: Now and in the future
State-of-the-art models, in situ measurements, and available satellite observations are all required to adequately characterize short- and long-term physical dynamics associated with ENSO and Pacific decadal variability. Seasonal-to-interannual forecasting of the ecosystem response to ENSO in the CCS and throughout the northeast Pacific will depend on our understanding of how interannual climate variability alters ocean biogeochemistry and productivity at the base of the food web, and therefore how predictive models should be modified to capture the dynamic range introduced by these anomalous events. Unusual warm water anomalies, as observed during large ENSO events, may serve as important analogs for assessing the impacts of long-term warming on the pelagic ecosystem of the CCS. Regional simulations suggest similarities between the physical drivers leading to biogeochemical variability from ENSO and those in projected future upwelling systems (Rykaczewski and Dunne 2010). Further exploration of the mechanisms and predictive skill of forecasts on seasonal timescales will enhance our understanding and improve our projections further into the future. Global climate models are unable to anticipate anomalous warming events such as major ENSO events. As such, they are unable to detect large-scale events related to shifts in the distribution of pelagic species or track ecological changes associated with such events. Furthermore, the evaluation of model-based forecasts and projections of ecosystem variations and changes across timescales requires that long-term physical, biogeochemical, and ecological observation programs are maintained and others initiated. High-resolution modeling approaches for forecasts and projections should also be prioritized, so that ecosystem impacts of future climate anomalies can be anticipated and understood in greater detail.
Authors
Clarissa Anderson (Scripps Institution of Oceanography)
Samantha Siedlecki (University of Washington)
Cecile Rousseaux (NASA Goddard Space Flight Center, Universities Space Research Association)
Brian Powell (University of Hawaii, Manoa)
Bill Peterson (NOAA Northwest Fisheries Science Center)
Chris Edwards (University of California, Santa Cruz)
References
Bograd, S. J., & Lynn, R. J. (2001). Physical‐biological coupling in the California Current during the 1997–99 El Niño‐La Niña Cycle. Geophysical Research Letters, 28(2), 275-278.
Cane, M. A., S. E. Zebiak, and S. C. Dolan, 1986: Experimental forecasts of El Niño. Nature 321, 827–832, doi:10.1038/321827a0.
Di Lorenzo, E., and Coauthors, 2008: North Pacific Gyre Oscillation links ocean climate and ecosystem change. Geophys. Res. Lett., 35, doi:10.1029/2007gl032838.
Du, X., W. Peterson, and L. O’Higgins, 2015: Interannual variations in phytoplankton community structure in the northern California Current during the upwelling seasons of 2001-2010. Mar. Ecol. Prog. Ser., 519, 75-87, doi: 10.3354/meps11097.
Fisher, J. L., W. T. Peterson, and R. Rykaczewski, 2015: The impact of El Niño events on the pelagic food chain in the northern California Current. Global Change Bio., 21, 4401-4414, doi:10.1111/gbc.13054.
Frischknecht, M., M. Münnich, and N. Gruber, 2016: Local atmospheric forcing driving an unexpected California Current System response during the 2015–2016 El Niño, Geophys. Res. Lett., 43, doi:10.1002/2016GL071316.
Jacox, M. G., J. Fiechter, A. M. Moore, and C. A. Edwards, 2015: ENSO and the California Current coastal upwelling response. J. Geophys. Res., 120, 1691–1702, doi: 10.1002/2014JC010650..
Jacox, M., E. L. Hazen, K. D. Zaba, D. L. Rudnick, C. A. Edwards, A. M. Moore, and S. J. Bograd, 2016: Impacts of the 2015–2016 El Niño on the California Current System: Early assessment and comparison to past events. Geophys. Res. Lett. 43, 7072-7080, doi:10.1002/2016GL069716.
Keister, J. E., E. Di Lorenzo, C. A. Morgan, V. Combes, and W. T. Peterson, 2011: Zooplankton species composition is linked to ocean transport in the Northern California Current. Glob. Change Bio., 17, 2498-2511, doi: 10.1111/j.1365-2486.2010.02383.x.
Liu, Z., and M. Alexander, 2007: Atmospheric bridge, oceanic tunnel, and global climatic teleconnections. Rev. Geophy., 45, doi: 10.1029/2005RG000172.
Mantua, N. J., S. R. Hare, Y. Zhang, J. M. Wallace, and R. C. Francis, 1997: A Pacific interdecadal climate oscillation with impacts on salmon production. Bull. Amer. Meteorol. Soc., 78, 1069–1079, doi: 10.1175/1520-0477(1997)078<1069:APICOW>2.0.CO;2.
McCabe, R. M., and Coauthors, 2016: An unprecedented coastwide toxic algal bloom linked to anomalous ocean conditions. Geoph. Res. Lett., 43, doi: 10.1002/2016GL070023.
McClatchie, S., A. R. Thompson, S. R. Alin, S. Siedlecki, W. Watson, and S. J. Bograd, 2016: The influence of Pacific Equatorial Water on fish diversity in the southern California Current System. J. Geophy. Res., 121, 6121-6136, doi: 10.1002/2016JC011672.
McKibben, S. M., W. Peterson, M. Wood, V. L. Trainer, M. Hunter, and A. E. White, 2017: Climatic regulation of the neurotoxin domoic acid. Proc. Nat. Acad. Sci., 114, 239-244, doi: 10.1073/pnas.1606798114.
Mckinley, G.A., and Coauthors, 2006: North Pacific carbon cycle response to climate variability on seasonal to decadal timescales. J. Geophy. Res., 111, doi: 10.1029/2005JC003173.
Nam, S., H. J. Kim, and W. Send, 2011: Amplification of hypoxic and acidic events by La Niña conditions on the continental shelf off California. Geophy. Res. Lett., 38, doi: 10.1029/2011GL049549.
Newman, M., G. P. Compo, and M. A. Alexander, 2003: ENSO-forced variability of the Pacific decadal oscillation. J. Climate, 16, 3853-3857, doi: 10.1175/1520-0442(2003)016<3853:EVOTPD>2.0.CO;2.
Peterson, J. O., C. A. Morgan, W. T. Peterson, and E. Di Lorenzo, 2013: Seasonal and interannual variation in the extent of hypoxia in the northern California Current from 1998–2012. Limnol. Oceanogr., 58, 2279-2292, doi: 10.4319/lo.2013.58.6.2279.
Rodgers, K. B., P. Friederichs, and M. Latif, 2004: Tropical Pacific decadal variability and its relation to decadal modulations of ENSO. J. Climate, 17, 3761-3774, doi: 10.1175/1520-0442(2004)017<3761:TPDVAI>2.0.CO;2.
Rykaczewski, R. R., and J. P. Dunne, 2010: Enhanced nutrient supply to the California Current Ecosystem with global warming and increased stratification in an earth system model. Geophy. Res. Lett., 37, doi: 10.1029/2010GL045019.
Sekula-Wood, E., C. Benitez-Nelson, S. Morton, C. Anderson, C. Burrell, and R. Thunell, 2011: Pseudo-nitzschia and domoic acid fluxes in Santa Barbara Basin (CA) from 1993 to 2008. Harmful Algae, 10, 567–575, doi: 10.1016/j.hal.2011.04.009.
Shipe, R. F., Passow, U., Brzezinski, M. A., Graham, W. M., Pak, D. K., Siegel, D. A., & Alldredge, A. L. (2002). Effects of the 1997–98 El Nino on seasonal variations in suspended and sinking particles in the Santa Barbara basin. Progress in Oceanography, 54(1), 105-127.
Shipe, R. F., and M. A. Brzezinski, 2003: Siliceous plankton dominate primary and new productivity during the onset of El Niño conditions in the Santa Barbara Basin, California. J. Mar. Sys., 42, 127-143, doi: 10.1016/S0924-7963(03)00071-X.
Siedlecki, S. A., N. S. Banas, K. A. Davis, S. Giddings, B. M. Hickey, P. MacCready, T. Connolly, and S. Geier, 2015: Seasonal and interannual oxygen variability on the Washington and Oregon continental shelves. J. Geophy. Res., 120. 608-633, doi: 10.1002/2014JC010254.
Siedlecki, S. A., I. C. Kaplan, A. J. Hermann, T. T. Nguyen, N. A. Bond, J. A. Newton, G. D. Williams, W. T. Peterson, S. R. Alin, and R. A. Feely, 2016: Experiments with seasonal forecasts of ocean conditions for the northern region of the California Current upwelling system. Sci. Rep., 6, 27203, doi: 10.1038/srep27203.
Valsala, V., S. Maksyutov, M. Telszewski, S. Nakaoka, Y. Nojiri, M. Ikeda, and R. Murtugudde, 2012: Climate impacts on the structures of the North Pacific air-sea CO2 flux variability. Biogeosci., 9, 477, doi: 10.5194/bg-9-477-2012.
Xiu, P., and F. Chai, 2014: Variability of oceanic carbon cycle in the North Pacific from seasonal to decadal scales. J. Geophy. Res.,119, 5270-5288, doi: 10.1002/2013JC009505.